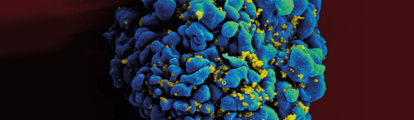
Finding HIV’s Achilles’ Heel
The human immunodeficiency virus has evaded the best efforts of vaccine developers for over three decades. And our own HIV vaccine has taken 15 years to make it into clinical trials. Here, I share why I believe we’re finally in with a chance.
I have been interested in HIV ever since my grad student days, having lost friends to the virus before effective drugs were available to keep the virus in check. Over 30 years, amazing progress has been made to life expectancy thanks to antiretroviral therapies, but an effective vaccine still eludes us. The NIAID has funded over 100 clinical trials of over 50 HIV vaccine candidates since 1987, but none have made it to licensure.
There are three main reasons that creating a vaccine for HIV has so far proved impossible. First, the virus changes very rapidly. In fact, the virus in your system a month after infection is very different from the one that you were initially infected with – it’s like hitting a moving target. Second, the virus has a number of other clever ways to evade the immune system (see "Evasive Maneuvers" on page 30). Third, HIV infects the very cells that your body uses to combat an infectious agent, namely CD4-positive T cells.
Several candidates have shown promise. In one of the highest-profile clinical trials for HIV to date, RV 144, promising early results generated cautious optimism with many investigators. Unfortunately, the response was not durable, providing only a 30 percent decrease in infection rates by the end of the study. The vaccine tested in RV 144 was based on a prime–boost strategy – with four injections of a vaccine using attenuated canary pox virus as a carrier to present HIV envelope proteins to the immune system plus two injections of a subunit vaccine, targeting a different HIV envelope protein (see "Vaccine Varieties").
Vaccine Varieties
Attenuated virus
A weakened version of a pathogenic virus, designed to infect cells and cause an immune response, but not harm the host. Examples: Measles, Influenza.
Subunit
A portion or protein of the pathogenic virus. Typically co-formulated with an adjuvant to excite the immune system. Example: Hepatitis B
DNA vaccine
A DNA plasmid encoding proteins from the pathogen is injected, to be taken up and expressed by the body’s own cells. Example: Only one DNA vaccine has been approved for human use (Japanese encephalitis).
Viral vector
A harmless virus is genetically engineered to express proteins from the target pathogen to provoke an immune response. Example: Profectus’ replication-competent vesiculovirus (VSV) vector. None approved for human use.
A chink in the armor?
The Profectus vaccine stems from an observation made by my colleague at the Institute of Human Virology – Tony DeVico – who noted that you can stop the virus from getting into the cell if you target sites that are normally hidden on the virus but become exposed when it engages its receptor. The first receptor the virus encounters is CD4, a cell-surface protein. The virus binds to CD4 using a “spike” made up of the viral envelope protein. Once bound, the viral protein changes shape to expose parts that engage a second receptor – this time a chemokine receptor (usually CCR5 or CXCR4). Only by binding to both receptors can the virus gain entry to the cell.
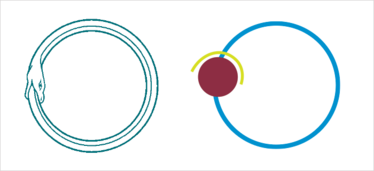
The FLSC vaccine is made up of sections of CD4 receptor (yellow) and its viral ligand GP120 (red), joined by a flexible linker (blue) that allows the two to bind, not unlike Jörmungandr, the snake from Norse mythology that circles the globe and eats its own tail.
To evade the immune system, the sequence of the HIV spike changes constantly, but the receptor-binding domains must remain constant to allow cell entry. The obvious target is the CD4 receptor-binding site, so why not target that? The answer can be found in the 3D structure of the envelope protein. The CD4 binding site is a deep cleft and obscured by loop structures and glycan groups, making it hard for the immune system to “see” and access the binding site. But when viral envelope protein binds to CD4 and undergoes its dramatic conformational change, other conserved areas are exposed and could be targets for the immune system. We realized that if we created an immunogen that looked like this altered spike protein, we could induce an immune response that would target the virus while it is in its transition state, before it enters the cell. The protein we produced consisted of a piece of CD4 and a piece of the envelope protein GP120, stitched together with a floppy linker that allowed the two to engage in such a way as to make GP120 change its shape. We call this transition state vaccine the full length single chain (FLSC).
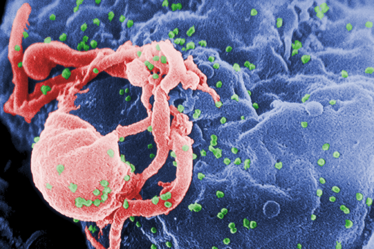
Profectus is born
The Institute of Human Virology was founded by Robert Gallo with two important goals: doing great basic research and translating this research into new therapies that can be developed into clinical products. Several years after I joined the Institute, I was asked if I would be interested in joining the spin-off company which would spearhead that translational mission – Profectus Biosciences. We had enough money from investors to launch the company – but not enough to move the vaccine into the clinic. I was doing the groundwork towards clinical translation, so I accepted the invite immediately. For me it was a no-brainer. I was not interested in becoming an academic and having to deal with all the rules that academics have to put up with. Since joining a small company, I have certainly spent a surprising amount of my time writing grant applications to help move our products into clinical trials. But there is a different mentality and way of working in a company that you really can’t transplant into an academic setting.
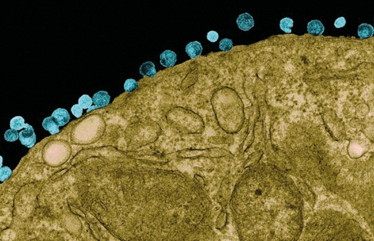
In the wilderness
We first described our protein construct back in 2000, so it’s taken us 15 years to bring it to Phase I clinical trial. Why the delay? It’s the usual story – not enough money. Even getting funding to do some preliminary studies in animals was a challenge. We had promising results in small animals. Next came studies in primates, the gold-standard model for HIV, to build up a solid database of evidence to prove that a vaccine was worth pursuing. In the meantime, we were looking for money to develop a product we could take into clinical trials. This took us quite some time. It is frustrating because with the right funding we could have brought the vaccine to the clinic years ago – the vaccine really hasn’t changed that much from the very first experiments we published. We’ve made a lot of variations on it for experimental purposes, but the principal construct has remained the same.
The breakthrough in funding finally came when the Bill & Melinda Gates Foundation, along with the Henry Jackson Foundation, stepped in to put a significant sum behind the vaccine. Concurrently, Profectus was able to get a Small Business Innovative Research grant (SBIR) from the National Institute of Allergy and Infectious Diseases. A classic case of “feast or famine” – we were in the financial desert for a long time and then all of a sudden the stars aligned and we’ve been able to move forward rapidly to the point of entering clinical trials.
Into the clinic
We have now started enrolling the Phase I trial, and the first participants, healthy 22–45 year olds, have received the vaccine. At this stage, we’re solely interested in safety; in other words, does the vaccine make anyone sick? Of course, once we establish safety, the million dollar question is “Will it work?” I’m confident that we will evoke an immune response but also well aware that we may need to fine-tune the precise formulation. In our primate studies, while a straightforward subunit vaccine generated a response, it wasn’t the 100 percent lifetime immunity that you see with some vaccines. To get the best protection, we also found that we had to induce the correct “immune balance”. This comes back to the fact that HIV targets CD4-positive T cells. If the vaccine induces the formation of too many of these CD4-positive T cells, it actually works in the virus’ favor. So the goal is to induce a predominantly antibody-based response, with just the right amount of T cells – it’s a fine line. It may be that the subunit vaccine, as currently formulated, is enough. But to be certain, we’re also testing a variety of different prime–boost regimens to expand the repertoire of immune responses being elicited by the vaccine; for instance, combinations of DNA and subunit vaccines as well as combinations with viral vectors such as the canarypox that was used in the RV 144 study. Once the subunit vaccine completes Phase I, we will initiate these additional trials to help us understand the best way to elicit the immune balance we need. We can learn a lot from animal studies, but ultimately you need human trials to be sure you have the balance right. My clinician friends regularly remind me that people are not monkeys – but, given our previous work in primates, I have every confidence that the vaccine will be safe and that it will generate the right immune response.
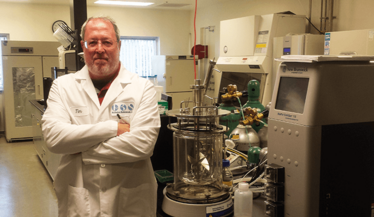
Timothy Fouts is Senior Director of Virology at Profectus Biosciences, Baltimore, MD, USA.
Evasive Maneuvers
HIV has a multitude of strategies to avoid detection and evade elimination by host defenses.
Shape shifting
A recent study reported that the mutation rate of HIV-1 in vivo is the highest of any biological entity, and significantly higher than previously reported (1). Mutations are created both by viral reverse transcriptase and by host cytidine deaminases. High genetic diversity benefits the virus by allowing it to dodge immune responses but a large number of mutations can cause inactivation, so the virus walks a fine line.
Invisibility cloak
Reverse transcription of viral RNA into double-stranded DNA normally triggers innate pattern recognition receptors. The capsid protein that surrounds the HIV-1 genome prevents infected macrophages from recognizing and destroying the virus, by binding to specific host factors. By using drugs to suppress the host factors involved, researchers have been able to “uncloak” the virus and prevent replication – one avenue for future antiretroviral therapies (2).
Going to ground
While modern antiretroviral therapy allows patients to live largely normal lives, the disease is never truly wiped out. Instead, the virus hides out in cells and tissues protected from the immune system – ready to stage a re-invasion if treatment is interrupted. It was thought that the virus remained dormant during this tactical retreat, but recent research suggests that the virus continues to replicate and evolve within the lymphoid tissue, even in patients with undetectable levels of viral RNA in blood samples (3).
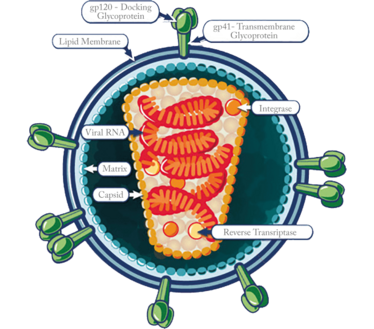
- JM Cuevas et al., “Extremely high mutation rate of HIV-1 in vivo”, PLoS Biol. 13, e1002251 (2015).
- J Rasaiyaah et al., “HIV-1 evades innate immune recognition through specific cofactor recruitment”, Nature 503, 402–405 (2013).
- R Lorenzo-Redondo et al., “Persistent HIV-1 replication maintains the tissue reservoir during therapy”, Nature, 530, 51–56 (2016).
Timothy Fouts is Senior Director of Virology at Profectus Biosciences, Baltimore, MD, USA.