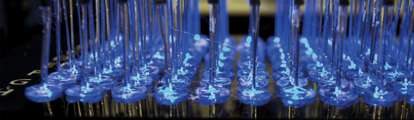
The Impact of COMET
A new approach to epigenetic control may allow precise spatiotemporal targeting of disease-controlling mechanisms.
The more we learn about epigenetics, the more we see its involvement in a plethora of human diseases. Gene dysregulation plays a role in cancers, neurodegenerative diseases, developmental disorders, and more. But the epigenome presents a challenge – how do we address its failings in diseased tissues without disrupting the careful balance of regulation in healthy ones? Our current methods aren’t sufficient; the few epigenetic drugs that have received approval treat the whole body, risking epigenetic changes in undamaged tissues. Even targeted approaches are difficult, as most require the introduction of a genetic modification before they can be applied. But what if we had a way to precisely control epigenetic gene regulation using nothing but small molecules and visible light? We propose a new approach known as COMET that might provide exactly that.
Purposeful precision
Epigenetic regulation, a critical means of controlling DNA in the human genome, is integral to health and disease. What exactly does it entail? It’s a collection of highly dynamic processes involving multiple chromatin modifying and remodeling enzymes. Those enzymes control access to genes and their expression through the covalent modification of DNA and its associated histone proteins. Developing precise tools to control the epigenome has been of great interest in basic research for some time – and it’s gaining increasing attention from the clinical side of the equation as we begin to grasp the widespread potential application of epigenetic therapies for disorders ranging from cancer to Alzheimer’s disease.
We refer to the general technique we’ve developed as “chemical optoepigenetics.” What does that mean? Chemical refers to our use of small molecules to modulate the epigenome, and opto- means that we use light (photons) to control the inhibitory activity of those small molecules. As you can probably guess, the technique relies on optically controllable small molecules that target a class of epigenetic regulatory enzymes called histone deacetylases (HDACs). We refer to these small molecule inhibitors as COMET (Chemo-Optical Modulation of Epigenetically regulated Transcription) probes and use them to provide high-resolution control of epigenetic mechanisms (1).
Having precise spatial and temporal control of epigenetic mechanisms – without the need for genetic modification – opens up new avenues to dissect the dynamic process of HDAC-mediated genome control. Optogenetic methods using light-responsive ion channels have transformed neuroscience, and we now also have genetically encoded, light-inducible transcriptional effectors. But both of those methodologies require prior delivery of genes into the target cells. Our “all-pharmacological” approach to controlling gene expression is a major advance for the field. That’s not to say that there were no pharmacological options before COMET – but current chemicals in epigenetics, for instance those that reversibly modify the N-terminal tails of histone proteins, are limited in terms of their selectivity and our ability to control them. COMET probes will allow more precise targeting of dynamic chromatin modifications, potentially at subcellular resolution. We hope that will eventually lead to a better understanding of the direct and so-called “off-target” effects of small molecules.
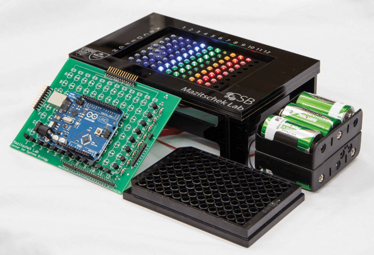
The LED array assembly used in the Mazitschek laboratory to conduct a COMET assay. The instrument can be seen in action at go.nature.com/1rrsKDe
COMET in the clinic
There’s still optimization work to be done before we can start looking at COMET for clinical applications. One day, though, our probes may translate into novel therapeutic strategies that make use of conditional and selective epigenome modulation. So far, it’s oncology that has seen the greatest advances in epigenetic therapies. Even now, we have epigenetic drugs like vorinostat and romidepsin on the market and many others in clinical development – but none of them can be precisely controlled. Outside oncology, an area of growing interest is the application of epigenetic therapies to a range of brain disorders, including neurodegeneration found in Alzheimer’s disease and affective disorders like depression or bipolar disorder. Exciting preclinical proof-of-concept studies with non-optically controllable HDAC inhibitors already exist, and those provide us with an impetus for exploring the use of COMET probes in the same context. The added precision we can bring to the table in such a complex tissue as the brain may confer additional advantages. Other, more accessible tissues like blood, the retina, and the ear might also see significant benefits from that kind of precise control. And of course, not having to genetically modify the targeted tissue to obtain optical control of a biological process has a number of distinct advantages.
Ultimately, we’d like to see a wide range of disorders benefit from COMET, including cancer and nervous system disorders that involve focal dysregulation of epigenetic mechanisms in specific regions or tissues. Being able to control where and when an epigenetic therapy is applied could conceivably overcome many of our existing approaches’ shortcomings – for instance, the fact that current epigenetic treatments expose the entire body to an active drug, whereas we would provide a system for careful targeting.
COMET technology allows researchers to devise and implement new types of epigenetic studies that could not be contemplated previously. They can control the epigenome of a single neuron within a complex circuit without having to first genetically modify the cell. They can create a dose-response gradient within a single tissue or tumor sample. And we encourage them to come up with new ideas! The Arduino-based, microprocessor-controlled LED array platform we developed is inexpensive and readily available, which we hope will increase the rate at which investigators discover novel photochromic probes and optimize their use.
An optoepigenetic future
All this is only the tip of the optoepigenetic iceberg. Our COMET concept can be applied to a wide range of epigenetic mechanisms beyond HDACs, including other epigenetic “writers” and “erasers,” like the proteins involved in histone methylation and demethylation, or “readers” like those that bind to sites of chromatin modification. Each strategy will require the development of novel chemical probes with appropriate binding kinetics and selectivity profiles. As part of this, we’re exploring other photochromic scaffolds with improved optical properties for use in vivo in tissues where photon scattering (deflection when a particle hits molecules larger in size) and absorption can be minimized through the use of longer wavelengths of light. Current versions of our COMET probes can be turned on with light, and we’re investigating the alternative possibility of optical control that allows them to be turned off instead. Of course, while we explore these new concepts, we’re also advancing our HDAC COMET probes toward clinical translation. It’s our hope that a step up in simplicity and precision control could lead to a new day for epigenetic research – and, eventually, patient care.
Stephen J. Haggarty is Associate Professor of Neurology at Harvard Medical School, Associate Neuroscientist at Massachusetts General Hospital’s Center for Human Genetic Research, and the Director of the Massachusetts General Hospital, Chemical Neurobiology, Boston, USA.
Ralph Mazitschek is Assistant Professor at Harvard Medical School, Co-Director of the Chemical Biology Platform at the Center for Systems Biology at Massachusetts General Hospital, and Assistant Professor in the Department of Immunology and Infectious Diseases at the Harvard T.H. Chan School of Public Health, Boston, USA.
To enable commercialization of this technology, the inventors have filed for a patent (WO 2014160221) on the novel compositions and methods of treatment, and are currently seeking licensing arrangements for application for therapeutic development.
- SA Reis et al., “Light-controlled modulation of gene expression by chemical optoepigenetic probes”, Nat Chem Biol, 12, 317–323 (2016). PMID: 26974814.
Ralph Mazitschek is Assistant Professor at Harvard Medical School, Co-Director of the Chemical Biology Platform at the Center for Systems Biology at Massachusetts General Hospital, and Assistant Professor in the Department of Immunology and Infectious Diseases at the Harvard T.H. Chan School of Public Health.
Stephen J. Haggarty is Associate Professor of Neurology at Harvard Medical School, Associate Neuroscientist at Massachusetts General Hospital’s Center for Human Genetic Research, and the Director of the Massachusetts General Hospital, Chemical Neurobiology.