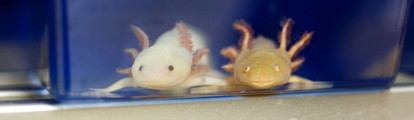
Going out on a (Regrown) Limb
Meet the researchers uncovering the secrets of tissue regeneration.
We humble humans oft admire the seemingly miraculous regenerative power of certain curious creatures – the starfish, the salamander, the sea cucumber… What scientist hasn’t dreamed of unlocking such amazing therapeutic potential? Here, we meet two groups of researchers who are delving into the molecules behind the “miracles.”
The amazing axolotl
Capable of regrowing lost limbs, repairing damaged internal structures, and, perhaps most remarkably, reconnecting severed nervous structures, the axolotl is regarded by many as the king of the regenerative kingdom (1). But are they really that different? “Axolotls have virtually the same genes as mammals,” says Karen Echeverri, Associate Scientist, Marine Biological Laboratory, Woods Hole, USA. “The key difference seems to be that, early on in wound repair, axolotl cells migrate to repair lesions, whereas mammalian cells appear to exhibit early programming to form scar tissue.”
Echeverri is co-author of a paper that outlines a new pathway, which is responsible – at least in part – for the pro-regenerative response of axolotl glial cells to spinal cord damage (2). Though the underlying components of injury response are the same in axolotls and humans, the process is different. “In axolotls, we’ve observed that the gene c-fos is transiently upregulated following damage to the spinal cord – for about 24 hours – after injury, whereas we see sustained upregulation in humans,” she says. “c-fos, which is an obligate heterodimer, chooses a different partner in axolotls and mammals, which completely changes the downstream genes that are affected.”
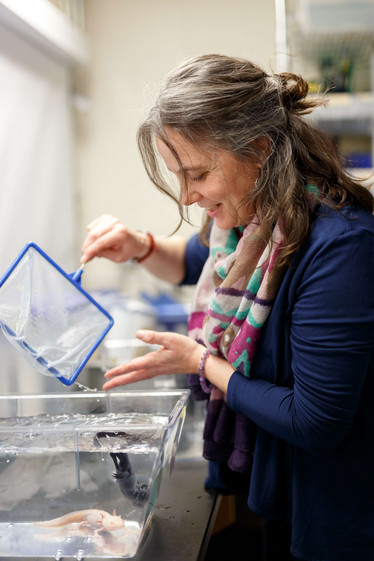
Karen Echeverri, working with the axolotl, the neotenic salamander capable of remarkable feats of tissue regeneration. Photo credit: Dee Sullivan, The Marine Biological Laboratory, Woods Hole, Massachusetts.
If the axolotl’s regenerative ability stems from a conserved evolutionary pathway, the big question is: how could the human process be manipulated with similar results? “Humans already have some level of regenerative capacity; for example, if part of the liver is amputated, then we are able to grow some of it back,” says Echeverri. But clearly, neural regeneration is much more complex. How do axolotls do it? “Their axons can actually regrow at the injury site. We know that in response to injury glial cells are activated to divide and migrate again – essentially becoming neural stem cells,” she says. “And if they can differentiate into new neurons, then they have to be able to be incorporated into existing neural networks.” As Echeverri digs deeper into process – aided by the publication of the axolotl genome (3) – she is driven by the major implications such knowledge could have in the neurodegenerative disease space. “If we can understand how the axolotl guides this process at a molecular level, then we can begin to translate that into treatments for patients,” she says.
One important aspect of the research was the team’s apparent ability to disturb regenerative capacity through inhibition of miR-200a, highlighting its important role in creating a pro-regenerative environment. Having discovered how to “break” the process in the axolotl, Echeverri hopes to move a step closer to translation by achieving the opposite in human cells. “That’ll be the next major step – seeing whether we can do this in human cells in vitro. Can we create a more permissive environment for regeneration?”
The magic mouse
Elsewhere, researchers have been exploring a far less studied organism: the spiny mouse – a mammal with several tricks up its sleeve (4). “We’ve found that the spiny mouse is capable of regenerating everything we’ve so far explored – full thickness skin, cardiomyocytes, cartilage, skeletal muscle (5), and, in some very promising but as yet unpublished preliminary studies, spinal cord tissue,” says Malcolm Maden, a Professor of Biology at the University of Florida.
Keen to find out more, the team has been further unraveling the mouse’s secrets. “We believe that the spiny mouse has a completely different immunological response to wounding,” Maden says. “It does not lay down fibrotic molecules associated with scarring because it does not localize collagen promoting cytokines into the wound,” he explains (6, 7). According to Maden, the ubiquitous nature of this process across several tissue types is cause for optimism. “If we can find some common mechanism behind all these systems, then the short-term goal is to make a lab mouse that can regenerate its tissue,” he says.
Maden admits he hasn’t given translation into the clinic too much thought, but believes there’s huge potential. “Whilst many companies have had little success developing anti-fibrotic molecules, we might be able to contribute positively to that endeavor,” he says. Moreover, he’s confident that the requisite technology exists. “You can imagine some kind of anti-fibrotic antibody that could be delivered intravenously. You could use exosomes, or even secretomes, which people are now testing in rats and mice.”
Maden concedes that many challenges – not all scientific – lie ahead. “The field is full of interesting molecules designed to inhibit or knockdown certain aspects of developmental pathways. The problem is that, with so many pressing medical demands, ideas like regenerating skin are not that high up the list of priorities. Instead, focusing on something like a stroke or heart attack, which is more medically relevant, could be the key to unlocking regenerative potential.”
Echeverri concludes with the harsh reality and a key question: “Tissue regeneration is a very complex process. Have we lost that ability or have we selected against it for a repair pathway to protect ourselves from infection or to conserve energy?” The answer may allow us to truly understand how far the field of regenerative medicine can progress.
- BJ Haas and JL Whited, “Advances in Decoding Axolotl Limb Regeneration”, Trends Genet, 33, 553-565 (2017). PMID: 28648452.
- KZ Sabin et al., “AP-1[cFos/JunB]/miR-200a regulate the pro-regenerative glial cell response during axolotl spinal cord regeneration”, Commun Biol, eCollection (2019). PMID: 30854483.
- S Nowoshilow et al., “The axolotl genome and the evolution of key tissue formation regulators”, Nature, 554, 50-55 (2018). PMID: 29364872.
- AW Seifert et al., “Skin shedding and tissue regeneration in African spiny mice (Acomys)”, Nature, 489, 561-565 (2012). PMID: 23018966.
- JO Brant et al., “Cellular events during scar-free skin regeneration in the spiny mouse, Acomys”, Wound Repair Regen, 24, 75-88 (2016). PMID: 26606280.
- M Maden et al., “Perfect chronic skeletal muscle regeneration in adult spiny mouse, Acomys cahrinus”, Sci Rep, 8, 8920 (2018). PMID: 29892004.
- M Maden & JO Brant..“Insights into the regeneration of skin from Acomys, the spiny mouse”, Exp Dermatol, [Epub ahead of print] (2018) PMID: 30457673.
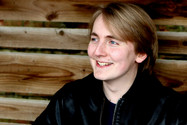